09 March 2024
Quantum Computing Unlocks the Future of Technology.
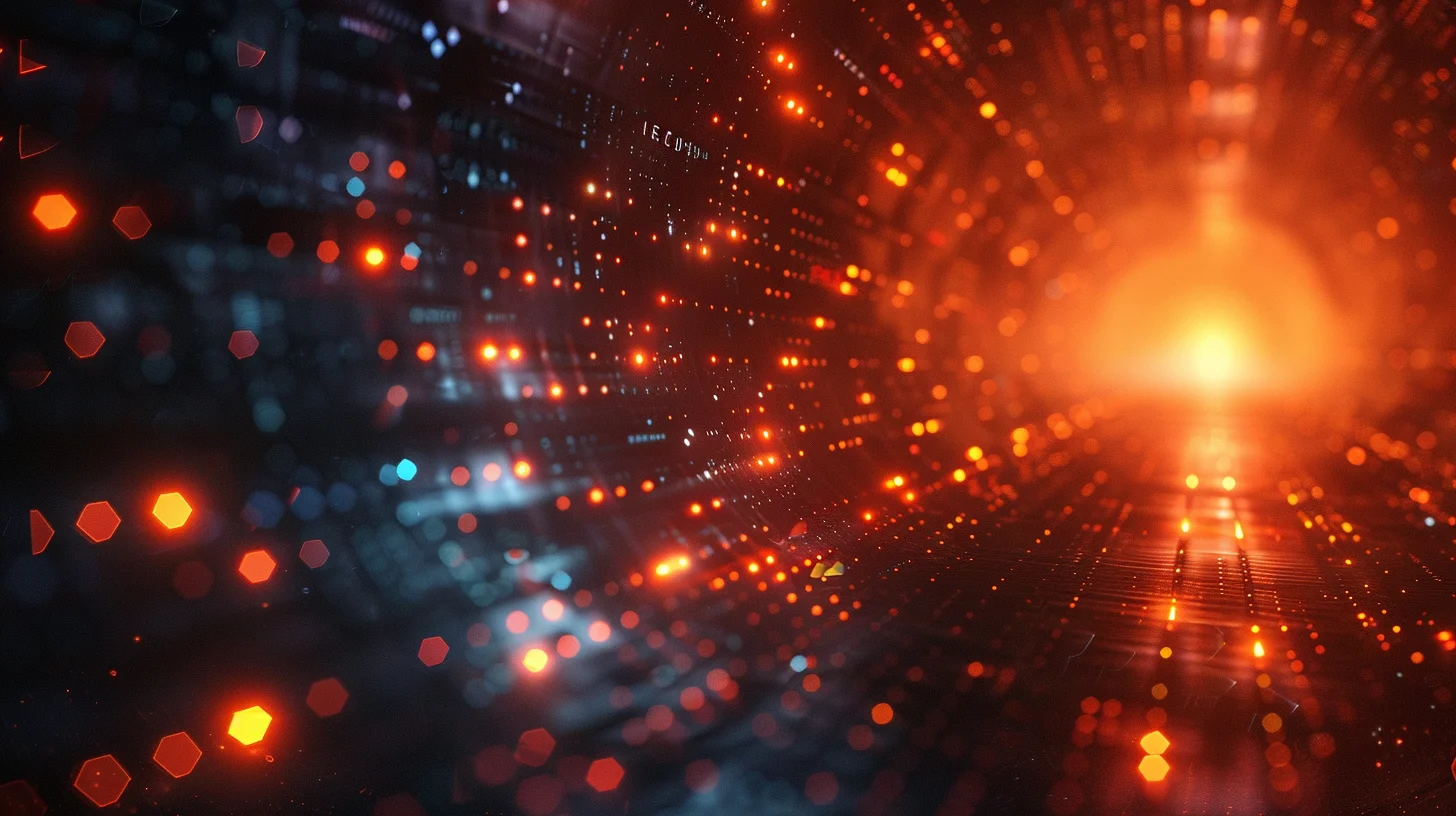
Press the play button in the top right corner to listen to the article
Quantum computing represents a revolutionary approach to computation, leveraging the enigmatic principles of quantum mechanics to process information in ways fundamentally different from traditional computing. This burgeoning field holds the promise of solving complex problems that are currently beyond the reach of classical computers, including drug discovery, cryptographic security, financial modeling, and climate research. In this article, we delve into the intricate world of quantum computing, exploring its foundational concepts, technological advancements, and the challenges that lie ahead in its development.
At the heart of quantum computing lies the concept of the quantum bit or qubit, the quantum counterpart to the classical binary bit. Unlike a classical bit, which can exist in a state of either 0 or 1, a qubit can exist in a state of 0, 1, or any quantum superposition of these states. This property, known as superposition, allows a quantum computer with n qubits to represent and process 2^n different states simultaneously, offering an exponential growth in computational power with each added qubit.
Another cornerstone of quantum computing is entanglement, a quantum phenomenon where qubits become interconnected in such a way that the state of one (no matter how far apart they are) can instantaneously affect the state of another. This phenomenon underpins the quantum computer's ability to perform complex calculations more efficiently than its classical counterpart, as entangled qubits can be used to perform parallel computations.
Quantum computing also leverages quantum interference, which involves the manipulation of the probability amplitudes of qubit states to reinforce or diminish certain computational paths. This principle is exploited in quantum algorithms to eliminate incorrect paths and enhance the probability of arriving at the correct solution, further boosting computational efficiency.
Developing quantum computers, however, presents formidable technical challenges. Qubits are notoriously sensitive to their environment; even minor perturbations can cause decoherence, whereby the qubits lose their quantum mechanical properties. Maintaining the coherence of qubits requires sophisticated error correction techniques and ultra-low temperatures, typically achieved using dilution refrigerators to cool the qubits to near absolute zero.
Quantum error correction is another critical area of research, addressing the issue of errors that arise during computation due to decoherence and other quantum noise. Unlike classical error correction, which can directly measure and correct bits, quantum error correction must navigate the no-cloning theorem of quantum mechanics, which prohibits copying an unknown quantum state. This challenge has led to the development of intricate error correction codes that protect the integrity of quantum information without violating the principles of quantum mechanics.
Despite these challenges, significant advancements have been made in the field of quantum computing. Various physical systems are being explored as potential qubit candidates, including trapped ions, superconducting circuits, and topological qubits. Each platform has its unique advantages and technical hurdles, from the high coherence times of trapped ions to the scalability of superconducting circuits.
Among the most promising developments in quantum computing is the advent of quantum supremacy, a term coined to describe a quantum computer's ability to perform a calculation that is practically impossible for a classical computer. In recent years, research teams have claimed to achieve quantum supremacy for specific, albeit contrived, problems, marking a significant milestone in the demonstration of quantum computational advantage.
Looking forward, the journey towards practical and scalable quantum computing is fraught with scientific and engineering challenges. However, the potential rewards are vast. Quantum computers could revolutionize fields such as cryptography, with quantum algorithms like Shor's algorithm offering the capability to break widely used cryptographic protocols, necessitating the development of quantum-resistant cryptography. In material science and chemistry, quantum simulations could unlock new understandings of molecular structures and reactions, paving the way for novel drugs and materials.
In conclusion, quantum computing stands at the cutting edge of computational science, promising to redefine what is computationally possible. While the path forward is complex and uncertain, the ongoing advancements in quantum algorithms, error correction, and qubit technology continue to push the boundaries of this exciting field. As researchers overcome the technical hurdles, the era of quantum computing will herald new possibilities across science, technology, and industry, unlocking computational capabilities hitherto deemed impossible.
The content, including articles, medical topics, and photographs, has been created exclusively using artificial intelligence (AI). While efforts are made for accuracy and relevance, we do not guarantee the completeness, timeliness, or validity of the content and assume no responsibility for any inaccuracies or omissions. Use of the content is at the user's own risk and is intended exclusively for informational purposes.
#botnews